van Oers, Pieter
Figure 1: Artist's impression of a black hole binary that has formed jets. Credit: Dana Berry (CfA/NASA) Most people have heard of black holes and know that they are objects so dense that not even light can escape from them. This suggests that nothing can be learned from a black hole using telescopes and satellite observatories, since we need to see them to study them, but the light within the grasp of a black hole could never reach us. While this means that we cannot probe what is inside a black hole, this does not apply to the surroundings of these compact objects. In fact, we are quite lucky that nature provides us with a means to indirectly study the black hole, that is, through the process of gas falling on to the black hole, a process called ‘accretion’.
Because of accretion we are able to observe black holes over many magnitudes in mass; from stellar mass black holes in our own Galaxy (in so called X-ray binaries; XRBs), to supermassive black holes (in Active Galactic Nuclei; AGN; possibly having a mass billions of times that of our sun) at the cores of distant galaxies (see Figure 2). In the stellar mass systems, the black hole is located in a binary system, accreting gas from an otherwise-normal companion star. Their extremely massive relatives in galaxy centres accrete interstellar matter or an occasional unfortunate star. In both cases, if matter has fallen prey to the enormous gravitational field of a black hole, it marks its demise with an extremely energetic and well-measurable death cry: it spirals inwards, forming an `accretion disc’ as it does so, which heats up through friction in the gas, until the resulting plasma is so hot it emits light, primarily in the ultraviolet in AGN, and in X-rays in XRBs, where the discs are even hotter. 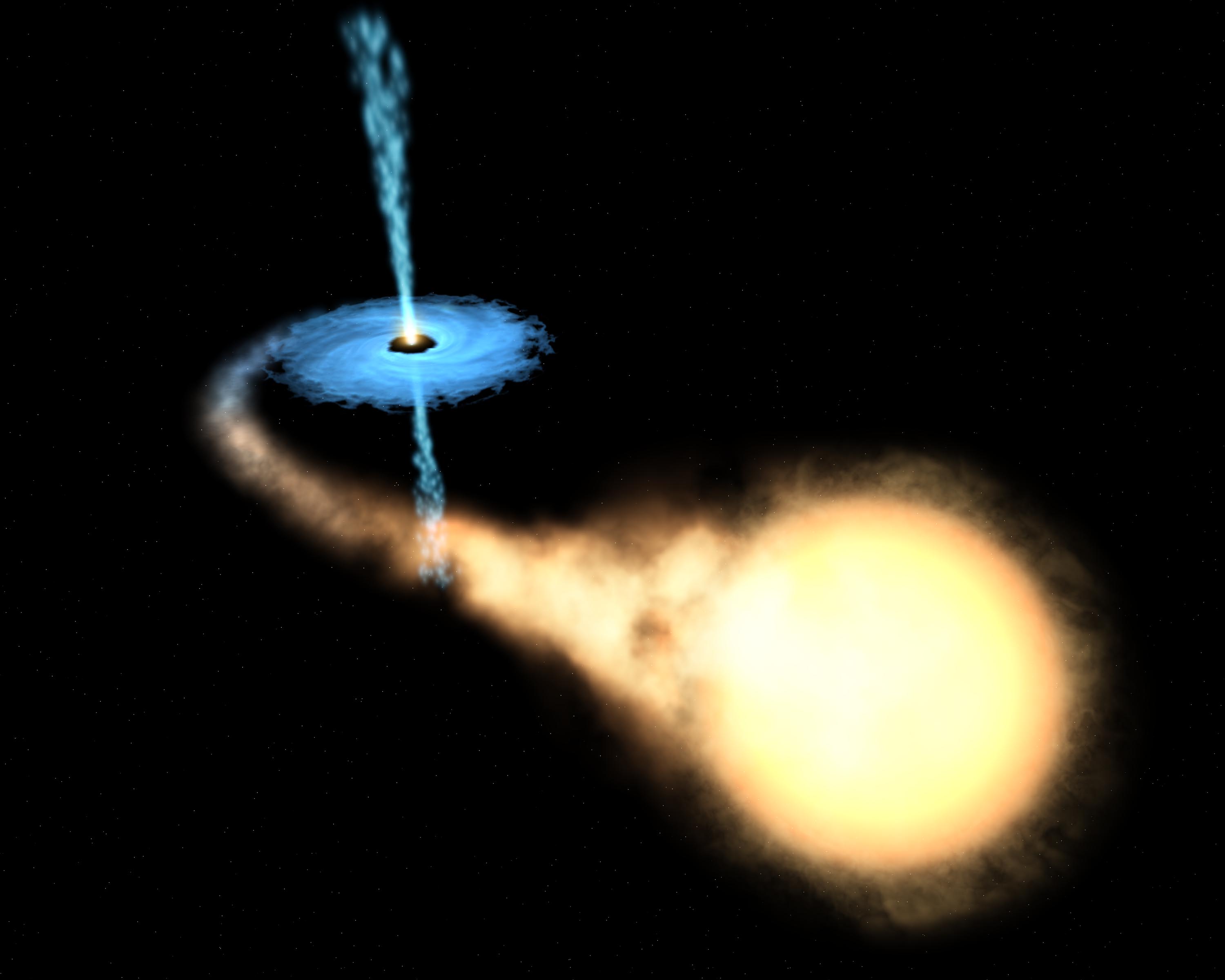 Figure 2: Radio observation of an AGN (Cyg A; left; Credit: NRAO/AUI) and an artist's impression of an XRB (right; Credit: Mirabel 2008, arXiv:0805.2378v2) Not only do we observe an inflow of matter in these systems. If the infall-rate of the plasma is too high, the accretor may not able to process all the matter and an outflow can be created (see Figure 3). This may be in the form of "bipolar plasma outflows", loosely termed "jets". These mysterious collimated flows still baffle scientists in terms of how they are created and what they are comprised of. The outflow need not be collimated, but can also occur in the form of a disk wind. There are indications that these forms of outflows complement each other: If the flow-rate of one component decreases the flow-rate of the other increases, ultimately balancing the total outflow. 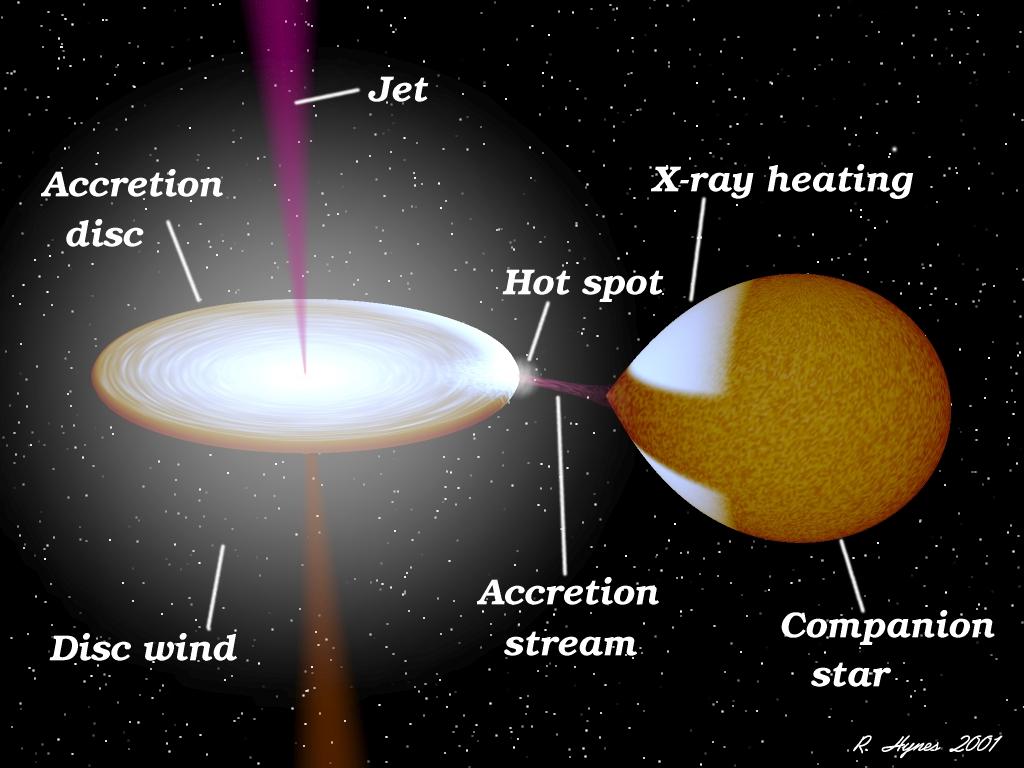 Figure 3: Schematic representation of a LMXB, depicting the companion star (right) and accretion flow (left). The latter consists of an inflow in the form of an accretion disk and outflows in the form of a relativistic jet and/or a disk wind. (Credit: NASA/Hynes 2001) The inflows and outflows are present in black hole systems of all masses. Although exact physical parameters, like the size of the system or the temperature of the disk, may vary with mass, we usually see a relatively simple mass-dependence of most parameters. This observation confirms the prediction from Einstein's theory of General Relativity that the physics related to black holes should be similar, regardless of mass. An important difference, however, between the "light-weight" and "heavy-weight" systems, is that the time required to observe changes in accreting black hole systems increases with mass. While changes in X-ray binaries are observed to happen on timescales from milliseconds to months, comparable variations can take up to millions of years for the most massive black holes in the centres of galaxies. But if the physics scales, perhaps we can learn about the supermassive black holes by studying their low-mass counterparts. If so, we may have a means to understand the supermassive sources within a human lifetime, or even over the course of a PhD! Figure 4: Typical soft (red) and hard (blue) X-ray spectra of an XRB. (Credit: Gierlinski et al. 1999) An extremely interesting feature we observe in X-rays when we look at the binaries, is that we generally find them in several distinct spectral states. Two of these are really important: The "soft" state and the "hard" state (see Figure 4). In the soft state we observe mostly low-energy `soft’ thermal radiation, coming from the accretion disc. In the hard state we observe mostly high energy `hard’ radiation, coming from the central part of the accretion flow, which could either be the inner part of the disk (the so-called "corona"), or the base of a jet.
Looking at AGN we observe several different types. We never see one type of AGN evolving into another because these changes would take too long. However, the different types of AGN could actually represent states, similar to the XRB spectral states. For this project we would like to find out if those AGN states map onto the XRB states if we account for mass-scaling. If not, perhaps some other physics, like the spin of a black hole, may be required to explain the observations. In the end these explorations will allow us to shed some light on AGN evolution over cosmic timescales.
|