Miskovicova, Ivica Black Holes and Binary Evolution The evolution of a star depends on its mass, but in any case, there is no escape from a final collapse. Mainly depending on its mass, a white dwarf, a neutron star or a black hole can be created (in general they are called compact objects in astrophysics). Black holes are created from the most massive stars, where masses of stellar remnants exceed 3 solar masses. Black holes are probably the most exciting objects in the Universe. They are infinitely dense singularities with infinitesimal volumes enclosed by the so-called event horizon. We cannot observe them directly, since the gravity inside the event horizon is that strong, that neither matter nor even light can escape its gravitational pull. This is why they are called "black holes". However, isolated objects in general are quite rare in the Universe. All the objects tend to join together into pairs or groups of few members orbiting around their common center of mass. The pairs of objects are usually called binary systems, or binaries. The evolution of such a binary is different to that of a single star. If a star expands in close binary, it can easily fill its so-called Roche lobe. Roche lobes are the surfaces separating the volume being bound gravitationally to one of the stars. When this happens, material from this star starts to flow to its companion via the inner Lagrange point of the binary system, where the gravitational forces from both objects are equal. The star which looses mass becomes the compact object after its collapse. When the companion star fills its Roche lobe, the interaction goes on again. In this case the compact object can grow by attracting matter from its surrounding. This process is called accretion. Since black holes produce their high luminosities exactly through accretion, we can detect them, observe them (indirectly), and especially, analyze properties of black hole binaries.
Accretion in X-ray Binaries Companion stars may be of different types and masses. According to these two characteristics, two different scenarios for accretion in X-ray binaries are known: 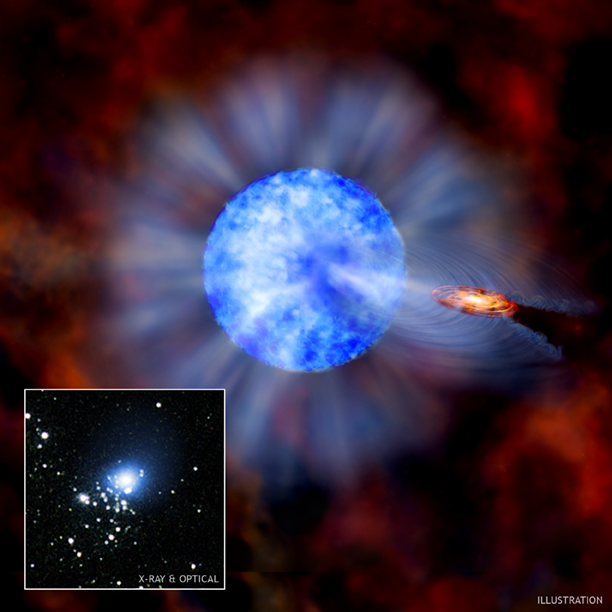
In Low-Mass X-Ray Binaries (LMXB) the companion star is of low mass. In this case, a stream of matter via Lagrange point is present in the system. Because the accreted material has a high angular momentum, it cannot fall directly onto the compact object. Instead of this, a large accretion disk is formed. In this disk the accreted material moves on circular orbits. Inner friction caused by the viscosity of the material heats the accreted gas such that it radiates away part of its energy and sinks deeper into the potential well of the black hole, until it falls through the event horizon. In High-Mass X-Ray Binaries (HMXB) the companion is a high mass star. Masses of several tens of solar masses are common in these systems. Such stars have strong stellar winds carrying away large amounts of material from these stars. The shape of the wind is strongly influenced by the gravity of the compact object, such that it is asymmetric and that there is a part of the wind, which is focused onto the compact object. Compact object can then feed off the stellar wind in these systems. This process, called Bondi-Hoyle-accretion, results in much smaller accretion disks, as the wind does not carry much angular momentum. However, the mass accretion rate is still high enough to produce very luminous X-rays (several 10000 solar luminosities are typical). Illustration of the stellar wind in the X-ray binary can be seen in the picture on the right (Credit: Illustration: NASA/CXC/M.Weiss; X-ray: NASA/CXC/CfA/P.Plucinsky et al.; Optical: NASA/STScI/SDSU/J.Orosz et al.). In this project we analyze both binaries with black holes or neutron stars as a compact object. The behavior of accreting neutron stars should be similar to the behavior of accreting black holes, except that they have a solid surface and stronger magnetic fields. The aim of the project is to study High-Mass X-Ray Binaries in greater detail. As mentioned before, accreting binaries are extremely luminuous. They emit X-ray photons with very short wavelength and very high energy. With such an energy, the accreted material close to the black hole can be photoionized. When X-ray photons pass through the medium close to the black hole, they interact with atoms and can transfer energy to the electrons, which are bound within these atoms. When this happens, electrons are able to escape from atoms. The process is called photoionization. The only condition is, that the energy of the photon must be higher than the energy by which the electron is bound, which is fulfilled for high-energy X-rays. 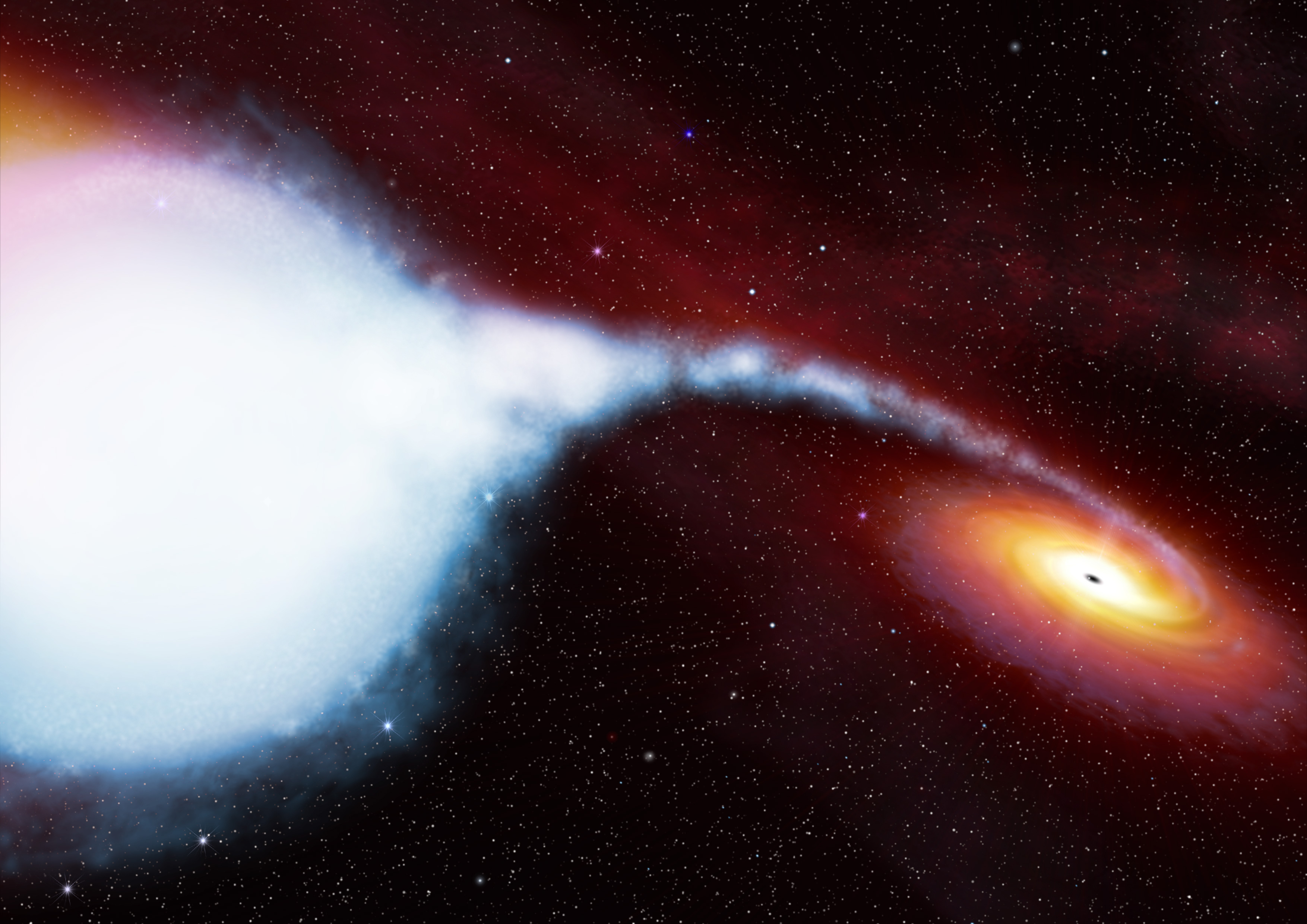 Investigating the wind properties We study spectra of X-ray binaries, i.e., the number of detected photons as a function of the energy. Due to photoionization, photons are absorbed in the medium around black hole. Thus, we detect less photons and observe so-called absorption lines at certain energies in the spectra of these systems.  These lines are important for our study, as they give us information about the properties of the matter close to the black hole, such as its ionization state or abundances of chemical elements in this material. Moreover, we can use observations of the black hole at different positions on its orbit around the companion star, allowing us to determine properties of the wider environment around the black hole, like the density distribution or the velocity profile of the stellar wind. To obtain this information, we need to perform a precise analysis of the spectra. We use observations from Chandra and XMM-Newton satellites, which orbit the Earth. Only spectra obtained by transmission grating detectors aboard these satellites are sufficient for such an analysis. The spectral analysis itself lies in fitting these spectra with already existing numerical models based on theoretical knowledge of photoionization processes.
Understanding all these properties is important for several reasons. For example, detecting accretion disk winds and other outflow phenomena like outbursts, which are also marginally included in this project, is only one possible approach to study these parameters. At the end we can compare them with parameters inferred from other types of analyses. This provides an independent test for accuracy of different types of analyses. Parameters, obtained from our analysis are basics and they are necessary for black hole research, since most of the further predictions of processes close to the black hole strongly depend on these properties .
|